
Climate Institute
September 2016
Authored by:
Rachel Levine, M.S.C.E., Chief Engineer
Dwight Macomber, PhD E.E., Research Consultant
Executive Summary
Dr. Sandy MacDonald et al. (2016) have recently called for the construction of a high voltage direct current (HVDC) electrical distribution network in their publication entitled Future Cost-Competitive Electricity Systems and their Impact on US CO2 Emissions. This proposal has been formed into an initiative known as the North American Supergrid (NAS), which calls for a mostly-underground HVDC overlay network interconnecting the three existing alternative current grid systems in the continental United States. They demonstrate that the large land area of the contiguous forty-eight states has sufficient diversity in meteorological conditions to ensure that much the moment-to-moment demand for electrical energy can be obtained from renewable wind and solar sources without energy storage capacity, resulting in an up to 78% reduction in power sector carbon emissions. Simulations indicate that this network would enable renewable energy to be transmitted to areas that don’t have sources of renewable energy enabling far greater use of carbon neutral sources. The result would be a significant reduction in overall CO2 emissions at electric bill rates that are competitive with those of present markets. The NAS presents numerous technical challenges spanning several areas of study. This report illustrates the major complications that will most likely be encountered throughout the planning and installation processes of this mostly-underground system and provides insight into how installation contractors can best address these problems.
This reports outlines and elaborates on crucial design aspects and makes important conclusions about the NAS’s environmental impacts. Variations in the natural landscape have a clear effect on the hypothetical construction of the national grid. Soil properties are perhaps the greatest limitation that designers and construction teams face. Should they use native soils to surround cable and backfill trenches, they must maintain critical moisture values to ensure proper heat dissipation. Similarly, natural hydraulic systems (namely water migration patterns) must be maintained despite soil changes. The location of bedrock also causes monetary, time, and water quality concerns. Blasting bedrock to lay cables is a costly and time consuming task and, when done incorrectly, can cause temporary water quality concerns.
The proposed underground HVDC grid would be best implemented by building a nodal bipolar system coupled with voltage source converter (VSC) stations. This configuration enables maximum resilience and flexibility in the bi-directional transportation of renewably-sourced electricity with minimal environmental and mechanical interferences. Because the electric and magnetic fields originating from transmission cables are static, any negative effects on human or animal health originating from either field type can be considered negligible. A similar trend was found with mechanical interferences with one exception: possible minor impacts on the migratory capabilities of flightless land mammals and/or insects in the immediate vicinity of a cable right-of-way stretch, due to weak magnetic fields originating from the transmission line. Magnetic directional compasses may also become slightly disoriented from this effect. While the underground system configuration does not directly reduce the impact of such fields, cables themselves will be far less susceptible to an electromagnetic pulse (EMP) attack or extreme weather event (a valuable safeguard not afforded by above-ground lines). The overall conclusion of this report is that, while surveyors must use caution to minimize environmental impacts, the negative impacts derived from the implementation of an underground HVDC grid are insignificant compared to the benefits that it would achieve regarding national security and carbon reduction.
Introduction
The Climate Institute has partnered with Dr. Sandy MacDonald to propose the creation of a primarily underground integrated HVDC grid for electricity distribution in America’s contiguous states, a proposal entitled the North American Supergrid (NAS). If implemented, our proposal would enable the efficient and stable transmission of carbon neutral energy over long distances. This report will summarize the technical challenges associated with our proposal offering analysis and recommendations addressing these concerns. Throughout the body of this report, material is organized by the overarching scientific discipline to which it most closely relates.
Electromagnetic Physics
The proposed grid relies on an efficient and flexible long-distance electrical transmission network system. Present-day semiconductors and control technologies would enable the movement of large amounts of electrical energy from areas with mass renewable generation capacity to areas with insufficient capacity on short notice.
HVDC transmission technology is most appropriate for this objective due to its high efficiency for long-distance transmission, while also providing crucial security and resilience properties.
Bipolar vs. Monopolar Transmittance of Electricity
A “HVDC overlay” is created by interconnecting existing AC (alternating current) distribution networks to a new DC (direct current) transference network. A network of point-to point HVDC links would interconnect thirty-two major points on the existing AC grid (otherwise known as “regional market areas). For maximum network flexibility, each HVDC link in the network overlay would be capable of transmitting energy in both directions. Employing agile voltage-source converters (VSC) at each end of the HVDC links that interconnect the HVAC nodes facilitates bi-directional transmission.
To construct a mostly underground system, other in-ground constituents, such as native soil constituents and human-made structures, must be considered. Existing below-ground infrastructure, including gas, oil, and water pipelines, often employ cathodic protection systems to reduce corrosion on their metal surfaces (a cathodic protection system applies a negative potential to the metallic structure to be protected). This is balanced by the positive potential applied via a grounded anode, which then supports a small current. Buried monopolar and bipolar (earth-neutral) HVDC systems can interfere with proper operation of these protection systems if any ground current associated with the HVDC facility overwhelms the protection current of the utility. This can most easily occur for monopolar HVDC systems. During normal operation, bipolar systems carry all transmission currents on conductors which are isolated from the ground. No currents that might interfere with cathodic systems are impressed into the earth. This is a principal justification for employing bipolar HVDC systems in congested areas. Additionally, balance control circuitry in modern HVDC systems is able to limit any imbalance between bipolar conductors and the interference field to a fraction of a percent of the current.
To date, most bipolar HVDC transmission systems are designed to move energy in one direction only from one specific location to another. In the event of a fault, unless the line is shut down, current that was carried by the faulty conductor is routed via a monopolar earth-return. Extended operation under this fault condition compromises nearby utilities.
The proposed bi-directional HVDC grid offers redundancy throughout the system by providing alternate routing paths. In the event of a failure of one bidirectional link during repairs, energy may be geographically re-routed around the fault through alternate links pathways. When a fault on the bipolar HVDC overlay system first occurs, there are voltages momentarily induced on adjacent utilities by the transient currents generated by the fault. These disturbances have been the subject of one study and have been found to be of little or no concern. Thus, adjacent utilities in shared rights-of-way remain protected during and after the occurrence of faults in the HVDC overlay network. Therefore, as long as electricity is rerouted in the case of faults, it is possible to bury cables near underground pipelines.
Capacity Limitations and Converter Technology
The methodology of the MacDonald, et al. study required that electricity demand is met “for every hour to every market while operating within current technology limits.” It included installation of an HVDC transmission network that can transmit electrical energy over large distances more efficiently and at lower cost than HVAC technology. The simulations assumed a 32-node network, with nodes placed across the continent at regional market areas (RMA) presently served by their own HVAC grids. Transmission capacity for the interconnecting HVDC lines was constrained to be no more than 12 GW. A 12-GW line would be composed of two parallel 6-GW lines, the limit assumed for present technology. These and possibly other levels of HVDC power operating capacity may alter the specific technologies chosen for the various links. At each network node, a two-way connection is made between the local HVAC tie-point and one or more links in the proposed HVDC grid overlay. In the case of excess power demand on the local AC grid, a voltage-source converter (VSC) converts DC energy on the HVDC grid to the proper voltage, frequency and phase to support the local AC demand. If the renewable generation capacity in the RMA is able to support local demand, the VSC passes through any energy arriving at the node from an any HVDC link in the overlay to where energy is required.
Employing VSCs at the network nodes provides additional capability for stabilization of the existing HVAC grid. Not only can VSCs be dynamically controlled to change the direction of energy flow between the HVDC and the HVAC grids as required for renewable energy redistribution.
As a result of general increases in energy demand and the growing penetration of distributed renewable generators, principally wind and solar, the interconnects and many of the HVAC RMAs have become less stable. As a remedy, VSCs can be employed to stabilize operation of weak HVAC systems through control of the reactive power on the AC side.
Proposed Cable Hardware
When determining the design and installation of this grid, the properties of the HVDC cable (particularly the cable’s exterior polyethylene casing and copper core) must be considered to ensure that unnecessary corrosion and wear do not occur over the system’s lifetime. Figure 1 shows the construction of a typical HVDC triple extruded cable, which would serve as the model cable for this entire project. Due to its construction, only the outer “PE sheath” will be in contact with the natural immediate surroundings (soils will be scrutinized with this in mind). While the immediate surroundings of the cable sometimes consist of a manmade silica fill (especially when native soil is not favorable), existing soils still have the potential to interact with the cable due to their use as backfill material, and therefore surrounding soil composition must still be considered during this installation task.
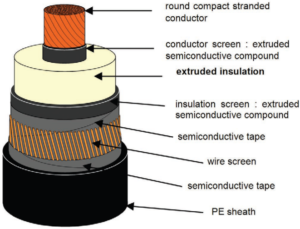
Figure 1: Common layers found in an extruded Cross-Linked Polyethylene (XLPE) HVDC cable
EMP Protection
In large part, the electrical power transmission grid is made up of a series of many long and highly conductive metallic cables. As long conductors are supported in open air on insulators, the cables also act as large antennas which intercept low-frequency (long-wavelength) electromagnetic waves or electromagnetic disturbances present in the atmosphere.
Solar flares, through the ejection of solar coronal mass, can lead to rapid drastic changes in the Earth’s magnetic field. These geomagnetic storms can induce impulsive currents in bulk power systems around the globe. The currents, referred to as geo-magnetically induced currents (GICs), can be large enough to disrupt normal operation and possibly damage or destroy portions of bulk power systems. This is what happened in the 1989 geomagnetic storm that led to the collapse of the Hydro Quebec system. The sequence of events caused by geomagnetic storms has been thoroughly documented and studied. The economic costs associated with such events have been analyzed and the continuing risks assessed.
A serious human-made threat to the electrical transmission grid is the electromagnetic radiation generated by the detonation of a nuclear bomb. The series of impulsive electromagnetic waves caused by the nuclear reaction is referred to as an electromagnetic pulse (EMP). If the detonation occurs at an altitude of 30 km or greater, the scenario is referred to as high-altitude electromagnetic pulse (HEMP). The nature of this electromagnetic disturbance has long been studied, as it is a threat to many electrical and electronic systems, especially the utility grid. The three most significant portions of the HEMP electric field waveform are referred to as E1, E2, and E3, with E3 having the longest duration. Only E2 and E3 are of concern for the long conductors of the electrical utility grid, but the levels of E1 and E3 generated by a nuclear explosion can exceed those levels generated by natural processes. The HEMP threat is aggravated by the fact that the disturbance travels at the speed of light, exceeding the capability of conventional grid protection equipment to isolate the disturbance to a single region.
Overhead HV cables are directly exposed to the radiated electromagnetic pulse. Burial of the long conducting cables of a power grid reduces exposure to HEMP E3 in two ways: burying cables below the Earth’s surface places the partially reflective air-to-ground boundary between an incident EMP wave and buried electrical cables and the partially conductive soil further attenuates the EMP wave that propagates into the earth from the air-to-ground as it approaches the buried HVDC cable.
A second barrier for an EMP wave is provided by the cable itself. The center conductor of buried extruded XLPE high-voltage cables is surrounded by extruded XLPE insulation. This insulation is wrapped with a metallic screen which is further covered with an external insulating polymeric jacket. The construction of the buried cable is therefore electrically like that of a coaxial cable. Inside of a coaxial cable, the center conductor carries the signal and the concentric outer conductor is held at ground potential. The cable’s outer metallic screen intercepts and attenuates an impinging EMP wave before it reaches the current-carrying inner conductor. The effect of EMP on the cable (and the entire grid by extension) is therefore reduced.
The current (and voltage) induced on the outer metallic screen and the center conductor of a buried HVDC cable by an EMP waveform is, to the first order, dependent on the length of the conductor (longer conductors result in greater currents and voltages). Since the currents and voltages can be extreme and destructive, the outer screen of buried cables is physically “broken “at regular intervals to limit the induced currents. At these break points, one end of the electrically isolated cable screen section is grounded to the soil to provide an alternate path away from the center conductor for the EMP-induced current. The impulsive nature of EMP causes currents induced on the outer screen to couple energy into the center, utility load-bearing conductor of the HVDC cable. How much energy of the EMP wave is coupled into the center conductor is a complicated function of the EMP wave-shape and its angle of arrival, soil conductivity and burial depth, cable geometry and the length of the section of outer screen.
Based on technical and economic factors, a practical interval for the grounding of the outer conductive shield of buried lines is roughly two miles (3.2 km). The extent of the grounding arrangements required at the two-mile intervals is dependent on the soil conductivity and the effectiveness of the EMP suppression that is desired. Since these grounds are only effective during an extraordinary EMP event, they would not need to be on the scale of those designed for continuous use in a unipolar ground-return type system.
Soil Mechanics
Soil taxonomy is defined by several hierarchical classifications which designate soils based on their properties: Orders, Suborders, Great Groups, Subgroups, Families, and Series. As levels progress, categorization becomes more detailed and specific, with “orders” serving as the most general classification. Globally, there are twelve soil orders: Alfisols, Andisols, Aridisols, Entisols, Gelisols, Histosols, Inceptisols, Mollisols, Oxisols, Spodosols, Ultisols, and Vertisols. Each order possesses general properties that have the potential to interact negatively or positively with multiple electrical components of the underground network. Therefore, each must be carefully analyzed and assessed for risk potential. The chemical and physical tendencies of soils will largely contribute the longevity and overall efficiency of the HVDC system. While surveying must be completed to confirm proper cable placement, information about soils orders (coupled with knowledge of the field of soil mechanics) can provide a strong indication of which soils should be considered usable.
Physical and Chemical Soil Properties
The physical and chemical properties of soils will influence its interaction with human-made foreign materials. For the purposes of this analysis, a “physical” property will be defined as a trait that does not require manipulation of the soil’s natural state of matter to be expressed or measured, and includes areas such as electromagnetic properties, texture, and water content. While electrical resistivity and thermal tendencies of soil fall into this category, their extreme importance to proper functioning of the grid constitutes a separate detailed analysis (outlined in later subsections).
Conversely, “chemical” properties are evident only after or during a chemical reaction of some kind and include areas such as oxidized trace metal content, salinity, pH, and natural organic matter (NOM) content. Chemical properties mainly affect the longevity of the cable, which must be resilient to harsh conditions. The cable’s “PE sheath” was studied to determine the extent of this resilience. It was determined that high density polyethylene (PE) is not only extremely resistant to damage caused by water and saline, but also does not crack or scratch easily, a valuable safeguard against accidental or malicious tampering.
While high density polyethylene (HDPE) is not easily corroded, it is possible for it to succumb to damage when conditions favor strong oxidants and high temperatures. Depending on the exact thickness of the HDPE sample, it has the capability to withstand temperatures of up to 130°C. While this temperature is well above what natural conditions can produce, heat output from cables themselves could produce such excess heat. Constant monitoring of heat output and dissipation must be performed to maintain the rigidity of the PE outer sheath. To further explore the possibility of this occurring in practice, a thorough analysis of soil orders was completed to reveal several common naturally occurring trace metals that have the potential to be oxidized: Iron, Aluminum, and Magnesium. Of the common chemical derivatives produced from these compounds, none were found to possess strong corrosive capabilities towards HDPE.
Resistivity Potential
Soil resistivity is a measure of the electrical conductivity potential of a sample. When applying this principle to HVDC systems, it can be inferred that high soil resistivity will inhibit electricity flow and make complete and proper grounding difficult to accomplish, making it necessary to ensure that soil conditions along an HVDC line are favorable in order to promote system efficiency and machinery longevity. This is particularly important when considering the necessity of “backfilling” after grid construction (in which soil is placed over-top and/or around HVDC cables themselves). Trenches housing cables are often refilled with a maximum of 50% existing soil as an economical way prevent lasting ecological disturbance, as shown in Figure 2. While an exclusive study of soil properties (without field surveying thereafter) is at best a tentative approximation for resistivity, such analysis is nevertheless a promising guide as formal surveying takes place.
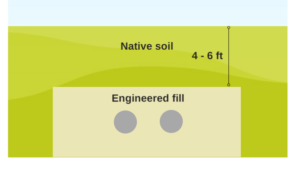
Figure 2: Cross section of a typical cable arrangement in native soil
Several previously discussed soil properties have a significant effect on resistivity values; namely, moisture content and overall soil texture/parent media. As moisture content increases, soil resistivity tends to decrease as chemical constituents become activated around conductors. For the purposes of HVDC application, the moisture content of the direct surroundings of the cable should be a minimum of 10%, as resistivity increases significantly when this value decreases.While moisture is clearly a critical component of usable soils, it should be noted that excessive moisture can cause concern in two forms. First, moisture can cause corrosion over time due to its potential to interact with other soil constituents, stimulating chemical reactions. Second, water logged soils are more likely to cause the formation of a frost layer, which can dramatically increase resistance (even if the host soil type is favorable during warmer seasons).
Resistivity is also strongly influenced by soil composition. Typically, soils which are low in electrolyte compound concentration (in the form of free ions) and moisture introduce the most resistance, solid stone and volcanically derived soils are prime examples of this phenomenon. An important exception to this rule is naturally occurring sand deposits which, when wet, possess high relative thermal conductivity compared to conventional favorable soils, such as clay. Depth to bedrock (discussed subsequently) and solid rock fragments present in the immediate area (above or below the electrode) must be accounted for during surveying.
Thermal Properties
Thermal resistance can be defined as the ability of a material to resist heat dissipation away from a heat source. Soils which are high in thermal conductivity (or promote heat flux in a given area and temperature gradient) tend to also display high moisture content due to the fact that air has a significantly lower thermal conductivity rating when compared to water. Water aids in increasing contact between individual soil particles, allowing heat to move easily flow across the gradient and away from the source. Soils rich in clay and sand tend to possess high thermal conductivity values compared to soils rich in NOM, which tend to “insulate” cables and prevent heat transfer to the ambient environment.
In order to properly determine if soil contains favorable thermal properties, thermal probing of field sites is often required. This information is necessary to construct a “soil thermal dry-out curve,” which relates soil moisture to its relative resistivity value. It is important to note that these curves often display a horizontal asymptote originating at the point of “critical moisture.” If the critical moisture value falls above this point, the lack of moisture will be further exacerbated by way of cable heat driving away the little moisture that is present. A lack of heat transfer between the HVDC cable and its surroundings can cause a dramatic increase in the temperature of the cable itself, ultimately causing thermal runaway (a cyclic process of heat output without subsequent dissipation) resulting in line failure.
This yields clues as to the behavior of a system during times of surplus moisture. As previously noted, the asymptotic behavior of a dry-out curve signifies that the critical moisture point has been reached and exceeded. Thereafter, the thermal resistivity will not reach zero, but simply trail along the asymptote as moisture continues to increase. This implies that excessive moisture does not cause an additional significant reduction in thermal resistivity after the critical moisture point is reached. When this information is coupled with the negative consequences of excess moisture outlined above, it is reasonable to conclude that excess moisture situations should be avoided, as they add no positive attributes to the system. Field testing should be completed to gain data for an accurate dry-out curve representation before grid installation.
Analysis of Soil Orders
Keeping in mind the extent to which the physical and chemical properties described above will influence system functioning, the 12 USDA soil orders will further be designated as usable or not usable. As noted above, classifications should be taken as a tentative conclusion until surveying is completed.
Alfisol – USABLE
Alfisols are a clay based soil usually derived from vegetation found in forests and/or savannahs in temperate environments. They contain a modest level of organics which grant some agricultural potential which can support a short growing season. Moisture content fluctuates heavily based on the season, allowing for the potential moisture to be high. Additionally, sublayers of this soil have the potential to contain oxidized iron or aluminum (which are usually absorbed to the clay itself) or other minerals such as quartz. This sublayer’s depth will dictate any resistive potential, as clay base itself has low resistivity.
Andisol – NOT USABLE
Andisols are a very specific soil order denoted by a primary composition which is made up of volcanic ash. It has the potential to have an extremely high mineral content, yet these minerals are usually non-crystalline (which is glass based and not metallic in nature). Soil has the ability to retain massive amounts of moisture due to the fact that it is not very densely packed, yet organic concentration varies based on location. Alloys (amorphous metals) can also be present in high quantities and act as excellent conductors (potentially interacting unpredictably with an HVDC system).
Aridisol – USABLE
Aridisol soils are often found in dry desert regions, and can best be described as sand based with poor moisture content and little to no organics. Sublayers usually contain silica, salt, gypsum, or calcium carbonates (all of which are generally poor conductors when compared to metals). Potentially high temperatures, coupled with very little moisture recharge, are slight concerns when the prospect of thermal runaway is considered due to the fact that thermal backfill will ultimately surround the cable itself. Built-in protections against this phenomenon within the HVDC cable itself must be evaluated to utilize this soil order.
Entisol – USABLE
Entisols are relatively under-developed soils that lack fully formed horizontal layers. Formation of this order is usually due to some form of disturbance, such as erosion, and is further inhibited by a very dry or very wet environment. The soil type is usually predominantly clay and sand, yet has no clear/predictable composition. Furthermore, a variety of materials can contribute to formation including dense rock, highly compacted soils, or even toxic waste. Moisture content can also vary widely, possibly compounding resistivity issues already present due to formation material. Location must be surveyed carefully before use.
Gelisol – NOT USABLE
Gelisol soils contain glaciated deposits characterized by a thick impenetrable permafrost layer (such as those found in tundra environments). They are key stores for organics, yet are only formed in very cold environments and therefore do not support the growth of most plants. This frost layer introduces resistivity concerns. Additionally, permafrost will most certainly hamper or halt trenching, and therefore should not be considered usable ground.
Histosol – NOT USABLE
Histosols are primarily composed of organics and are commonly found in peat rich bogs or swamp environments. Organics in the soil have a strong potential to oxidize other soil constituents, allowing for decomposition to happen rapidly due to the anaerobic conditions present when soil is completely immersed in liquid. Logistical construction problems could arise due to the soil’s inconsistency. Having a high concentration of organics, which act as heat insulation material, these soils should not be considered for cable ground.
Inceptisol – USABLE
Inceptisols form in a multitude of environments (much like closely related entisol soils), but possess some commonalities between formation locations. Ultimately inceptisols typically contain a larger than usual quantity of organics and moderate moisture retention ability. Minimal accumulation of oxidized metals, clay, and organics can occur, but rarely in amounts that exceed detection limits. This order is usually present in mountainous areas, but contains low erosion potential. Ultimate usage is dependent on the depth bedrock level in these mountainous areas.
Mollisol – USABLE
Mollisols are characterized by grassy vegetation followed by a layer of dark organically rich humus soil. They do tend to accumulate electrolytes, but are not hospitable for metallic compounds. They often receive and maintain high moisture levels, leading to saturation during some seasons. However, the lack of toxic or troublesome compounds mitigates this concern.
Oxisol – NOT USABLE
Oxisols are characterized by low nutrient/organic levels and a low concentration of electrolytes, but have a high concentration of oxidized metals. This is due to the fact that this soil is usually found in humid environments with a very acidic pH, leading to favorable oxidation conditions. They usually contain moisture but do not readily retain it. The extreme acidity of the environment could prove unfavorable for the PE outer sheath of the HVDC cable.
Spodosol – NOT USABLE
Spodosol soils contain three distinct layers; a rich organic surface topsoil, ash-based sublayer, and a reddish horizon layer (due to iron content). Generally, subsoils in this order have both low organic content and low clay content. Organics in the top layer have a strong potential to oxidize metals, which can flow down into the sublayers due to the fact that the base soil usually has a sandy texture with favorable permeability characteristics. Moisture content can vary, but tends to be high when rainfall exceeds evapotranspiration removal rates. The relatively uninhibited transference of problematic materials throughout the layers could pose issues for grounding equipment present along the length of the HVDC cable, and therefore should be avoided as a host environment.
Ultisol – USABLE
Ultisols are a mineral rich clay based soil containing mainly oxidized iron (due to a low pH environment) and quartz. The moisture content of ultisols varies widely based on formation location. Nevertheless, soil fertility is low due to a lack of organics and electrolytes, leading to a texture which promotes the mineral leaching throughout the soil horizons. Surface soils tends to be a more hospitable environment (and can even support the growth of forests), yet can still contain these troublesome minerals. Due to the shallow depth of cable trenches, it is reasonable that cables would most likely be laid in this more hospitable area.
Vertisol – NOT USABLE
Vertisols are a clay based soil (rich in calcium, magnesium, and lime) that responds dramatically by changes in water content by shrinking and swelling. Such activity can cause deep cracks in the soil layers in times of low moisture. In high moisture periods, water tends to settle in the topsoil of this order, and can even collect as standing water. This unpredictability will not only be troublesome during construction, but in turn causes issues with the thermal regulation of cables (particularly regarding heat dissipation), and should be avoided.
Should soils contain favorable properties as described above, it may be possible to bury cables in native soils directly, eliminating the use of cable sand entirely in certain line stretches. This is dependent upon the soil being clear of large rock fragments or other “unsuitable materials.” The possibility of utilizing native soils as fill materials must be evaluated on a case-by-case basis once cable routes are established (usually by means of surveying and moisture testing). Properties of native soil in such areas (described by soil orders) could possibly be used as a guide to pinpoint optimal sampling locations during the construction phase of the NAS.
Engineered Soils
As noted above, backfilling is a crucial component of system construction, as it is often cost effective and relatively simple to replace existing soils after in-ground cables have been placed. However, the area immediately surrounding the cable and its electrode may require an engineered soil fill to regulate and stabilize the thermal properties (even when the material itself is dry), a crucial component to the proper function of HVDC cables. Engineered soil parent material can vary based on the geographical source of the material relative to the construction site, but in many modern engineered fills (such as Fluidized Thermal Backfill) typical constituents include sand, cement, and aggregated minerals. When compared to native soils, engineered soils not only consistently achieve proper moisture levels, but also allow for proper compaction (without the possibility of soil contamination during the process).
It is vital to note that not all existing engineered soils can be applied to an HVDC system. For instance, typical highway construction methods require the presence of several engineered layers which are not conducive to grid construction. Two layers which are usually present underneath a paved road despite the expected load are the “base course” and “subbase.”
While the thickness of these layers is dependent on the quality of the native soil, both of these layers usually contain aggregates of high resistivity (such as granite) and overall diameter that are heavily compacted to form a single layer. However, use of highway right-of-way could still take place next to the paved surface in native soil, or along the highway median as shown in Figure 3.This presents a considerable advantage, as all locations containing major highways must be tested for soil quality and depth to bedrock before building, much like an HVDC grid.
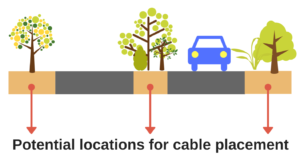
Figure 3: HVDC cables within a highway right-of-way
As opposed to highway support
materials, railroad subgrade is not as densely packed at the surface, as “ballasts” are made up of loose stones which are compacted (yet not cemented in place) to form a single layer. If necessary, it may be easier to insert HVDC lines directly below railway line surfaces in natural soils below (depending on their overall depth). This possibility must be explored further by installation contractors during the surveying process.
Geology and Topography
Natural surface characteristics of the Earth will greatly influence building of the NAS. Terrestrial and marine geological bedrock formations, as well as surface characteristics have the greatest potential to halt or slow construction.
Bedrock Location
According to ABB, a HVDC cable manufacturer, HVDC cables should be laid at a depth of 1.2 meters when possible. This requirement can be amended to a shallower depth if required by providing extra “mechanical protections,” presumably over the top of the structure. This 1.2 meter depth is both deep enough to maximize protection against accidental tampering, while also allowing for ease of access during cable maintenance.
While a shallow bedrock layer directly beneath a cable trench will not hinder thermal conductivity, the process of cutting through such a layer is time consuming, costly, and potentially environmentally hazardous. Bedrock is often removed by blasting, which can “fracture” nearby bedrock and cause geologic and/or potential temporary groundwater contamination (discussed subsequently). The increased time and extra equipment necessary to remove bedrock is another deterrent to using such environments.
In determining bedrock location, national low-resolution datasets are helpful to visualize areas which require further investigation due to the complexity of bedrock formations. Since the NAS will build upon existing rights-of-way, such geologic features will be crucial to identify (and avoid if possible) near highways and rail lines. It may be possible to obtain high resolution data near such transportation structures from local officials or individual construction companies, as depth-to-bedrock analysis is a necessary component of highway design.
Landscape
Underground lines must not come into contact with foreign objects in soil to ensure proper functioning and maintenance access, therefore the removal of trees directly on top of and immediately near a right-of-way stretch is essential (this ensures that roots will not become entangled in cable trenches or around cables). It is anticipated that tree removal will not be a large factor during cable installation, as this process has already been completed along highway medians or in highly developed areas. In virgin (or, previously undeveloped) lands, tree removal must be conducted with caution in order to ensure that the local biosphere traits are maintained. Ultimately, the usage of virgin lands should be avoided if possible. In other excavated stretches, it has been noted that native low-lying vegetation (such as grass) usually reforms within two years of initial installation. Should this occur, the continued development of these flora must be “managed” over time to ensure that these plants do not slowly invade the cable stretch itself.
Environmental Science
The installation of an underground HVDC system will undoubtedly cause the disturbance of the natural environments surrounding it due to initial construction and prolonged operation, especially if the ground into which the system is buried has not been previously developed. While the resilience of many biological systems will eventually restore balance in these areas, it is nevertheless crucial to examine the externalities associated with this new electric distribution system. In most cases, any negative environmental effects a HVDC grid might impose onto a system are coupled with subsequent positive effects. Certain complex areas within the realm of environmental science must be evaluated before a HVDC grid is built, including; ecological ramifications, potential hydrological changes, and air quality concerns. It is important to note that the degree of response to HVDC grid construction will vary by area, and how individual marine environments will respond (when compared to land systems).
Land-based Ecology
The positive and negative consequences of a HVDC grid will be discussed through the lens of these areas of study. It should be noted that this analysis is most representative of the possible effects of the development of virgin land. It is presumed that burial of cables along existing transportation avenues would not drastically change the ecologically disruptive tendencies already present.
Many modern infrastructure elements disrupt the ecosystem balances found in nature, particularly above ground power lines. Bird strikes, which occur when a bird runs into a power line during flight, has caused concern due to ensuing bird mortality and electrical system malfunctioning. Through an analysis of 14 individual studies, it has been estimated that at least 12 million birds are killed by above ground power lines yearly. Further compounding the issue, “approximately half of listed species (species on the Endangered Species List) have at least 80 percent of their habitat on non-federal lands and water, including areas used by electric companies.” The underground network that makes up the NAS will make important strides in protecting the bird population, and may also aid in the restoration of normal migratory flight patterns (as overhead lines will no longer emit magnetic fields throughout the majority of the grid distribution system).
Although heat will undoubtedly be dispensed from normal HVDC cable operation, surrounding microbial life will not be greatly affected by such “localized” change. However, the magnetic field output from cable operation could affect the migratory capabilities of land animals. Specifically, animals in contact with the ground surface (and residing up to 1.5 meters above the surface) will be susceptible to field output along the length of the line, as well as compasses used at such locations. Unless overhead lines are required, birds and airplanes would not be adversely effected by the presence of these low-lying fields (see Appendix A for calculations).
Marine-based Ecology
The prospect of placing cables in marine environments due to unsuitable land conditions presents unique environmental challenges. Cables must be able to withstand extreme pressure and saline conditions. Similarly, the surrounding environment must be able to rebound and thrive after cable installment is complete. The two main environmental issues surrounding marine HVDC cables are the release of chlorine gas and potential magnetic field distortion.
Chlorine gas is produced in submarine cables at the anode of a monopolar system (where the current comes into contact with the surrounding water) through the process of electrolysis. During ocean electrolysis, salt is dissolved to form positively charged sodium ions and negatively charged chloride ions. These ions then combine with electrons originating from the anode, as shown in the following reaction, to form gaseous chlorine50:
While chlorine gas is highly toxic and corrosive (especially in aqueous environments), it should be noted that it can only be produced in a monopolar HVDC configuration. The literature has noted that the use of bipolar systems virtually eliminates the risk of chlorine gas production in underwater HVDC systems.
Some concern has also been paid to the potential disorientation of aquatic organisms that may rely on the Earth’s magnetic field in order to migrate in the vicinity of HVDC cable. This issue of magnetic interference originating from underwater cables has been studied extensively, yet the literature has not reached a consensus as to the effects on migrating marine life (in part due to the mystery surrounding the mechanism that controls geomagnetic directional orientation in many land and aquatic organisms). During the installation of the SwePol HVDC link, some of these concerns were further investigated. The researchers concluded that the usage of a bipolar configuration significantly lowers magnetic field interference when compared to monopolar systems. Furthermore, shallow buried cables often only emit a strong magnetic field directly above the cable location, with some systems indicating a significant drop in magnetic field distortion at a horizontal distance greater than 5 meters from the cable.
While these promising results tend to lead to the conclusion that cables are generally safe for the surrounding oceanic environment, it is still prudent to take basic safeguards which further protect aquatic species. Namely, cables should be buried in the oceans’ dysphotic zone (at least 200 meters below the surface) or deeper whenever possible. In this location, photosynthesis cannot occur and sunlight diminishes rapidly as depth increases. This will minimize the risk to many endangered species and commonly consumed fishes, which usually reside close to the surface. Secondly, any protected coral reef formations must be mapped and carefully avoided during the installation process. While marine environments have been proven to successfully rebound after disturbances by cables, the fragility of many reef ecosystems
makes such resilience an unlikely trait.
Hydrology
The presence of groundwater and aquifer stores must be accounted for when designing an underground HVDC system. Not only does the construction of such a system have potential negative, albeit non-life-threatening and short term consequences for water quality, but the potential for permanent alteration of water flow through natural systems is also a possibility. Cable sand surrounding HVDC cables, as well as the heat derived from cable function, are the two most crucial system components to consider when evaluating hydraulic externalities. These components have the greatest potential to interact with aquifers and subsequent groundwater stores.
The contiguous 48 states house thousands of freshwater aquifers of varying degrees of drinkability. The geological and topographical characteristics of a location often determine the thickness of an aquifer, the depth to the water table, and the ease of access to the water supply. The distinction between confined and unconfined aquifers further complicates the compilation of data regarding groundwater depth and flow patterns. The depth to groundwater must be considered when building an HVDC system, as shallow water reserves may be unnecessarily disturbed by cable trenches (possibly causing standing water and/or privately held well interference). Therefore, it is vital to distinguish between the components of a groundwater system to accurately describe the data that will be used for mapping (Figure 4).
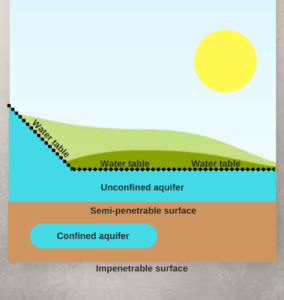
Figure 4: Groundwater formation cross section
A system’s “water table” designates the top of an aquifer (otherwise termed as “depth-to-water” in this document). Its depth is relative to the topsoil layer and can change based on variations in topography (even within a relatively small area). An aquifer is deemed unconfined if there is no presence of a bedrock/impenetrable layer above the water table. In such a case, a permeable soil layer is the aquifer’s only protection from the outside environment. As a means to determine the characteristics of major aquifers in the United States, USGS has classified several major aquifer systems as “principal aquifers,” which are considered extensive sources of drinkable freshwater (see Figure 5 in Appendix B). Analysis for the NAS research team will focus on these major aquifers when analyzing the depth-to-water regionally.
In a limited number of cases, the USGS provided a depth-to-water value for a principal aquifer. While these values are often determined through surveying techniques, the size of some aquifer formations implies that these figures are ultimately a regional guide. Construction of an HVDC line will require additional surveying along the route to confirm water table depths. Where a firm depth-to-water value was not given or could not be obtained through other sources, the minimum regional well depth necessary to produce water was used as a proxy value. While the casing of many potable wells often extends past the top of the water table, USGS notes that it is common practice to measure the depth to the water table in a shallow well as long as one accounts for the possibility of slight changes in the water table height based on the season. With the exception of the Floridian aquifer system and the New England aquifer system, a minimum depth-to-water value was obtained for all other principal aquifers, with the average depth totaling 11.6 meters from the surface for all continental principal aquifers.
While this value does imply that many HVDC cable trenches will not directly intersect the water table (since the cable depth will be maintained at 1.2 meters below the surface when possible), several important exceptions should be noted. While most principal aquifer systems with well-defined depth-to-water values exemplify this notion, the Denver Basin and Appalachian systems (named Valley and Ridge, Piedmont, and The Mesozoic Basin) have been proven to house very shallow aquifers. In such cases, special attention must be paid to survey of any rights-of-way intersecting these aquifers in order to prevent contamination or interruption of the water supply. In the case of the New England aquifer system, shallow groundwater supplies are also a concern, particularly close to the Atlantic coast. Due to the presence of shallow granite stores in this area (hindering HVDC construction), the impact of shallow aquifers on right-of-way usage will not be as pronounced as in other areas. Similarly, the aquifer formations in the Floridian system also exist at shallow layers, even causing standing water in some topographical areas, due to the unusually high rainfall levels in the state. Additionally, concerns with the swampy soil types that may be present in the area may constrain HVDC development. While high groundwater may indeed lower resistivity values, this tactic must be used with caution to prevent aquifer pollution.
It is possible for the depth of the water table and the type of aquifer formation to impact the properties of the native soil, which can in turn effect cable function. Generally, soils present above the groundwater table are more resistive to current flow, and exhibit higher water evaporation rates. This exacerbates the importance of the critical water level in the surface layers of native soil. While, in many cases, cables or thermal sand will not directly interact with groundwater formations, it can influence groundwater flow. This is particularly important when right-of-way routes are present near highly graded (or sloped) land. In these cases, “trench plugs” placed at the bottom of slopes can alleviate the possibility of water flow through cable sands preferentially causing disturbance of natural groundwater movement. In such high-risk areas, the possibility of eliminating the usage of cable sand entirely (if native soil is favorable) is the cheapest and arguably most effective protection against hydraulic disturbances.
Most aquifers in the contiguous 48 will not interfere with cable trenching and/or cable, even if trenches are above a confined or unconfined aquifer. Similarly, while temporary particulate contamination of unconfined aquifers may occur due to construction (especially if bedrock must be removed), trench plugs will ensure no permanent damage occurs to groundwater sources. In the vicinity of the aquifers located along the Eastern seaboard, as well as the Denver Basin system, surveying must be conducted with extreme caution to ensure no shallow water stores are disturbed, as this may create standing water or dramatically impact water flow (as water is more likely to flow through porous cable sand than through native soils in most cases). However, such minimal concerns are greatly outweighed by the benefits the NAS has the potential to provide, including the reduction of water withdrawals by at least 10% of 2015 levels (see Appendix C for calculations and data sources).
Air Quality
Throughout the construction and operation of HVDC transmission systems, air pollutants have the potential to be released. While conventional pollutants such as ozone and nitrogen oxides will undoubtedly be released during multiple phases of the HVDC transmission line’s lifetime, it is clear that such outputs are dwarfed in comparison to the enormous atmospheric carbon savings gleaned from increased penetration of renewables into the national electric grid. Nevertheless, potential air pollutants will be qualitatively and quantitatively evaluated based on the scope of this project.
Ozone output is a concern in above ground HVDC systems, especially those of negatively charged monopole configuration, due to its strong adverse health and environmental effects. In terrestrial HVDC transmission systems, most research concerning ozone output has been conducted using data from above ground configurations. Nevertheless, the results of this research still yield important clues about the behavior of underground systems. In all HVDC systems, ozone is generated from the system conductors along the transmission line, which can produce ozone and its precursors (such as nitrogen oxide). This phenomenon of “electrical discharge from the conductor” brought on by ionization is referred to as the “Corona Effect,” and influences how the transmission system as a whole interacts with the surrounding environment at many interfaces. The Corona Effect, and subsequent pollutant discharge, is dependent mainly on the environmental conditions the cable is exposed to. Thus, contact with moisture through precipitation or fog is the driving factor that determined the strength of the Corona Effect in overhead HVDC lines.
Scientific literature has proven that ozone concentrations specifically derived from overhead HVDC transmissions lines (with total voltages comparable to those proposed for the NAS are consistently below detection levels during times of favorable weather when compared to ambient ozone concentrations. Similarly, heavy precipitation events (assumed to be the “worst case scenario” for producing ozone emissions due to a subsequent inflammation of the Corona Effect) only produced ozone cloud concentrations totaling 0.01 ppm. Comparatively, 0.07 ppm is the maximum allowable daily limit of ozone exposure set by the United States National Ambient Air Quality Standards (NAAQS). In American megacities (such as Los Angeles), ozone levels are frequently recorded at 0.15 – 0.5 ppm levels, double the NAAQS value and a maximum of 50 times higher than ozone levels produced by HVDC technology.
The negative air quality effects of HVDC transmission (in the case of above ground cables) is clearly inert or ambiguous at best. Literature consensus has confirmed that environmental outputs from the lines themselves are inconsequential to the overall health of the natural system surrounding the cable. Due to a lack of implemented underground HVDC projects, there is little research detailing similar air quality impacts in these configurations. However, the fact that underground systems inherently contain an Earth barrier between cables and the outside world, as well as an insulation layer within the cable itself, implies that any similar emissions from the system would be emitted to the surrounding air at a minimal rate compared to overhead lines. Coupled with the fact that underground cables are protected from heavy precipitation and wind, the Corona Effect would seemingly also be diminished. Considering the immense fossil fuel emission savings achieved over the lifetime of the project, research indicates that The NAS would have a net positive contribution to air quality.
Public Health and System Interferences Due to Field Output
To date, the majority of studies attempting to quantify the health impacts of HVDC lines have been conducted using above-ground configurations. However, important lessons regarding the potential of HVDC lines to interact with humans, animals, and inanimate objects in their immediate surroundings can still be gleaned from such studies. In the case of underground lines, some negative effects may be avoided entirely due to burial. Additionally, it is crucial to illustrate the difference sources of magnetic fields and electric fields within an HVDC system. Electric fields are derived from the charge on conductors, while the magnetic field comes from current flowing through the conductors. For the purposes of this analysis, these two fields will be analyzed separately.
Static Electric and Magnetic Fields
When HVDC lines are operated without error, both the resulting electric and magnetic fields remain static in nature, meaning that they are constant with changes in time. More specifically, “the electrostatic (DC) field is a function of the voltage on the transmission line, and the magneto static (DC) field is a function of the current on the transmission line.”
Electrostatic fields created by HVDC transmission lines have been known to produce air ions via conductor operation, in the form of charged particles. There is very little research on such particles in relation to human health, and therefore there is no set limit for maximum recommended air ion exposure for humans or animals. Similarly, several studies involving the air ion output from above ground HVDC transmission lines concluded that there was no conclusive change in blood pressure, pulse, respiratory function, and body temperature due to air ion exposure. Indeed, car exhaust emits more air ions than a high voltage DC transmission line. The electrostatic field, although present, also does not have the capability to “penetrate an organism,” and therefore should not be a harmful component of this system.
It has been stated that of the fields outputted by HVDC line operation, only magnetic fields have any potential to affect biological systems. As such, it is crucial to put the magnetic field strength associated with overhead cables (and underground cables by extension) into context. The magnetic field of the Earth is less than 1 Gauss (G) in strength at the surface, while the magnetic field associated with HVDC lines has been measured at ±10% of this baseline along a given above-ground right-of-way. Comparatively, magnetic fields of 20 G in strength are required to impact the health of nearby animals or humans. Given these facts, the literature surmises that magnetic fields associated with HVDC outputs do not have the potential to impact cell growth or reproduction capabilities. From this research, it can be concluded that impacts on the migratory abilities of land-bound animals are the only health-based consequence of HVDC line operation. Calculations and mitigation techniques to quell these concerns can be found in Appendix A.
As prefaced by the analysis of electric field related health effects, such fields do not produce major mechanical interferences in other systems. Harmonic energy content and electric radio-frequency interference (RFI) that is shot into the HVDC side of the station converters may radiate and impair nearby radio and telephone communications. However, grounding the metallic screens of the buried cables greatly suppresses this interference. The capacitance between the center conductor and the screen wrapping serves to filter and suppress much of this high-frequency energy. Grounding the screen shields shunts these time-varying electric fields to ground. The grounding of the outer metallic screen of buried HVDC cables also serves to greatly suppress the radial voltage field of the high-voltage current-carrying center conductor. With the outer metallic screen held very near ground potential, the entire voltage drop (or rise) between the operating voltage of each cable and local ground is constrained to occur within the insulating layer within the cable. Therefore, no significant external DC voltage fields exist.
HVDC produced magnetic fields do have slight concerns as to potential interactions with unrelated man-made systems. As illustrated elsewhere in the report, large portion of the HVDC grid overlay will rely on buried cables. Aside from the reduced physical vulnerabilities of the network hardware, the proximity of the two buried cables results in a significant reduction in the net magnetic field at moderate distances from the pair. The opposing currents in the two conductors produce opposing magnetic fields, which then combine additively in the ground area between and above the cables, but oppose each other to partially cancel to either side of the burial trench. At a distance ten times the inter-cable spacing to either side of the trench, the static magnetic field is reduced to less than one-eleventh of that of a single cable.
Unfortunately, unlike the electrostatic fields produced by the system, harmonic energy content and radio-frequency interference (RFI) that is of magnetic nature and injected into the HVDC side of the station converters may radiate and impair nearby radio and telephone communications. No protection from magnetic interference is afforded by burying the cables. Specially designed line filtering may be required at the node locations to attenuate the RFI that is coupled to the HVDC transmission cables to suppress this interference. This would be determined by engineering analysis specific to the installations.
Final Recommendations
From this research, it is clear that actual soil data (obtained from either previously published geotechnical reports or sampling) must be used to paint a more accurate picture of environmentally viable right-of-way paths. While, arguably, most land stretches could become viable through bedrock blasting or soil amendments, the overarching goal of this project contradicts such actions. In order to construct the NAS in the shortest possible time span, cable trenching areas must be relatively favorable from the start to expedite the construction process.
Similarly, the prospect of burying some stretches of cable in sea beds along the United States coastlines presents another unique set of challenges, yet also provides a larger base of projects to research which have already accomplished this feat. Namely, some concerns with constructing such a system in United States occupied-waters include:
- Location of protected coral reefs
- Possible commercial fishing disturbances during construction
- Localized magnetic field disruption
- Placement near offshore oil rigs
These issues must be evaluated to ensure that marine life is protected throughout construction and operation, and that cables do not alter the environmental conditions of a marine area permanently.
Lastly, steps should be taken to establish wattage requirements for each HVDC transmission cable stretch. Vast differences in wattage (such as a transition between a link of 1 GW capacity and a link of 6 GW capacity) could impact the width of right-of-way required to properly space cables. This will also impact the thermal conductivity properties needed in such land stretches (as high capacity cables will ultimately emit more heat than those of lower capacity). Proper spacing ensures complete heat dissipation and will ultimately lengthen the life of the system. Total width requirements for each total GW capacity should be summarized in order to select the best right-of-way for every transmission path.
Downloadable pdf version with citations and appendixes available here.